Various types of cells that form our body all carry the same DNA information. From this identical blueprint, diverse types and quantities of RNA are copied, which are used to produce the necessary proteins that determine the characteristics of each cell. The amount of RNA produced from each gene is usually profiled as gene expression levels and is associated with cell-type-specific information, linking the diverse phenotypes of normal cells and diseases. Epigenome is the set of chemical modifications to the DNA that facilitate such cell-type-specific gene expression from the "same blueprint" of DNA. DNA is wrapped around small proteins known as histones and compactly organized within the nucleus as chromatin. Histones undergo various chemical modifications, including methylation, acetylation, and ubiquitination, and DNA itself can also be modified with methylation.
These modifications regulate the chromatin structure, making it more open or closed, which either promotes or suppresses transcriptional activity across different genomic regions. These “epigenomic modifications”, which are maintained in a plastic manner with DNA, play a critical role in gene expression control, enabling a wide variety of phenotypes in both cell-type-specific and disease-specific contexts.
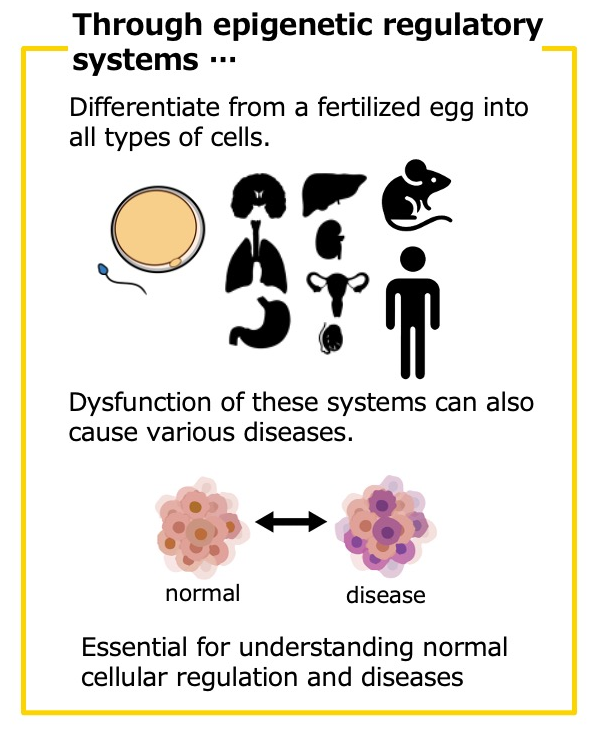
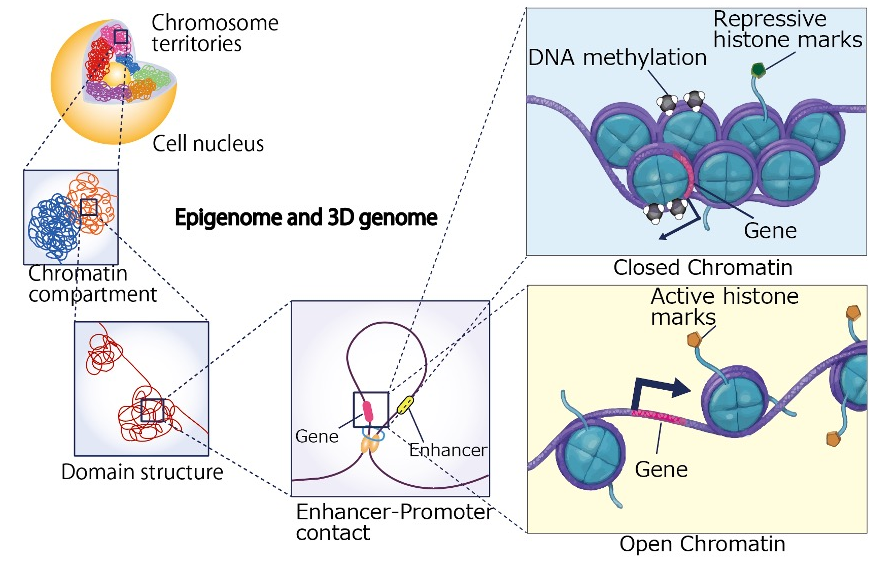
DNA stored within the nucleus as a chromatin structure consists of up to 3 billion base pairs in humans and mice. If the DNA from a single cell were stretched out, it would measure about 2 meters in length. However, it is not randomly packed inside the nucleus; instead, it is folded into an organized, three-dimensional structure (3D genome architecture). Importantly, most of our DNA consists of non-coding regions, which do not encode proteins. Nevertheless, these non-coding regions also carry various epigenomic modifications and play functional roles in regulating the transcription of many genes. However, even when we identify the epigenomic modifications present on these distal regions and predict them as active enhancers, it remains unclear which genes are regulated by these candidate enhancers. In this context, 3D genome information, including DNA loop structures that spatially connect enhancers and promoters, provides critical insights into understanding how non-coding regions are involved in gene activation.

We have been conducting research on epigenetic regulatory mechanisms and 3D genome architecture as a transcriptional regulatory machinery using the latest analytical methods, focusing on mouse germ cells and ES cells (Publications).
At RIKEN BRC, we will introduce and develop state-of-the-art techniques of bulk and single-cell gene expression analysis, as well as epigenome and 3D genome analysis. By fully utilizing these advanced analytical techniques and the extensive resources of model animals and iPS cell lines maintained by BRC, we aim to further explore gene expression regulatory mechanisms in reproduction, development, and diseases.
Development of new analytical techniques to obtain gene expression, epigenome, and 3D genome information, along with the establishment of advanced bulk and single-cell analysis platforms.
Using these analytical technologies to elucidate gene expression regulatory mechanisms in reproduction, development, and diseases contexts.
Obtaining comprehensive genomic data corresponding to the diverse phenotypes of bioresources and the construction of a database.
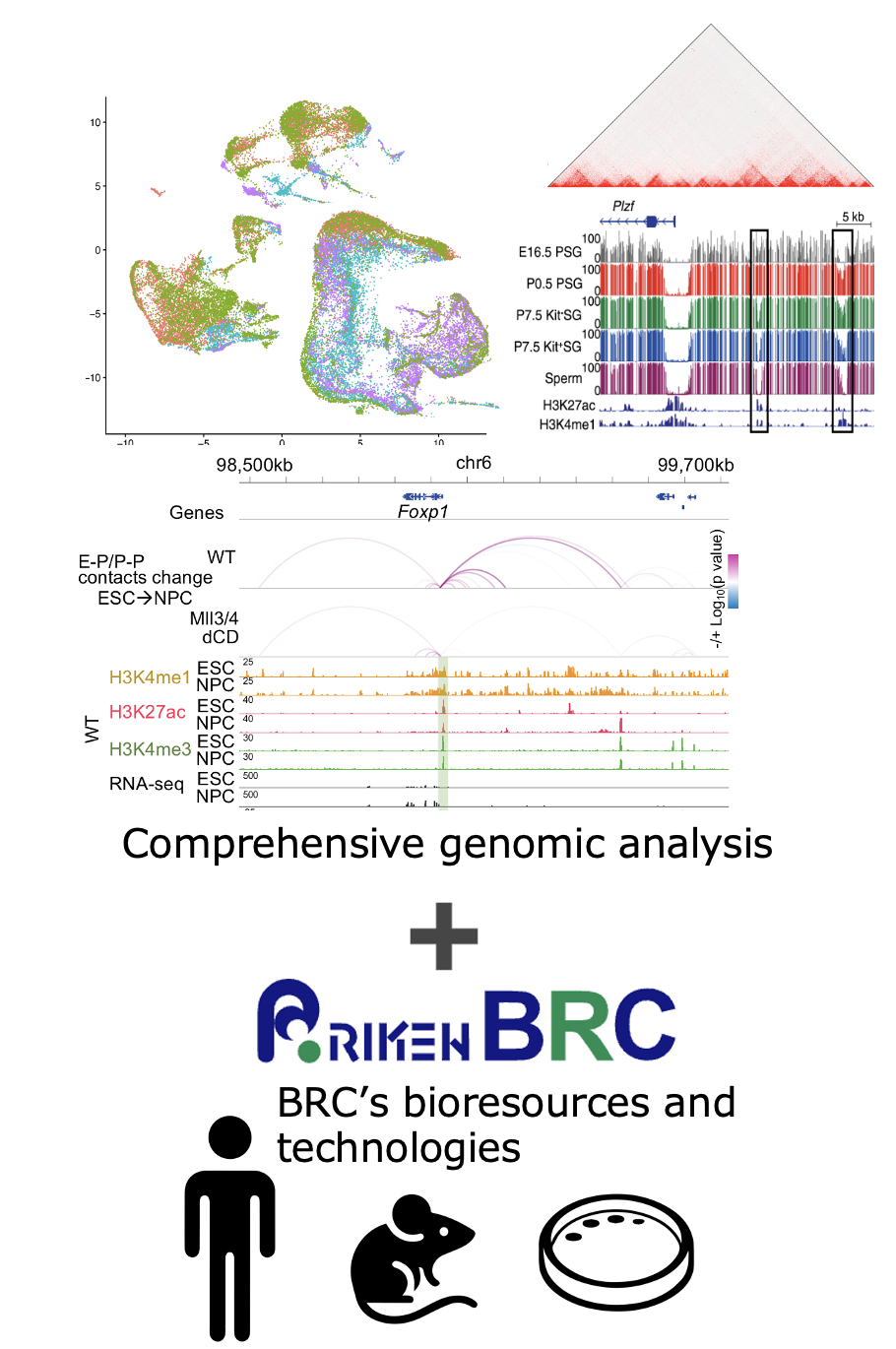